|
快速葉綠素?zé)晒?OJIP)可作為監(jiān)測植物在非生物脅迫下光合生理狀態(tài)的有效工具
歡迎點擊「漢莎科學(xué)儀器」↑關(guān)注我們! 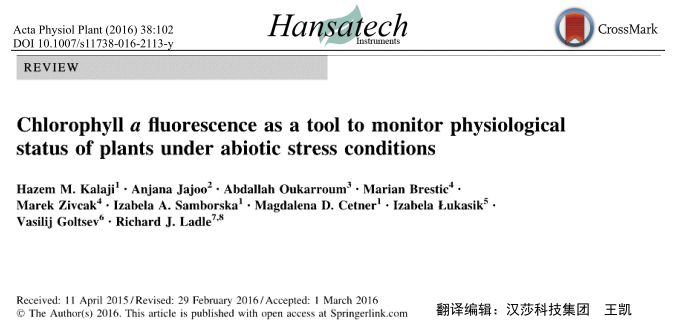
摘要在自然條件下生活的植物會受到許多干擾光合作用過程的不利因素的影響,導(dǎo)致生長、發(fā)育和產(chǎn)量的下降。葉綠素a熒光光譜(ChlF)的研究為葉片光化學(xué)效率研究提供了一條新的途徑。具體地說,對熒光信號的分析可獲取PSII反應(yīng)中心、捕光天線復(fù)合體以及PSII供體側(cè)/受體側(cè)的狀態(tài)和功能的詳細(xì)信息。在這里,我們回顧了快速ChlF技術(shù)(OJIP & JIP-test)分析光合反應(yīng)對環(huán)境脅迫的相關(guān)成果,并討論了這一創(chuàng)新方法的潛在科學(xué)和實際應(yīng)用。最近便攜式設(shè)備(Handy PEA & M-PEA, Hansatech Instruments)的出現(xiàn),特別是在作物表型分型和監(jiān)測方面,大大擴展了ChlF技術(shù)的潛在應(yīng)用。 關(guān)鍵詞 Chlorophyll fluorescence、JIP-test、Photosynthesis、Photosystem II、Quantum efficiency、Stress detection
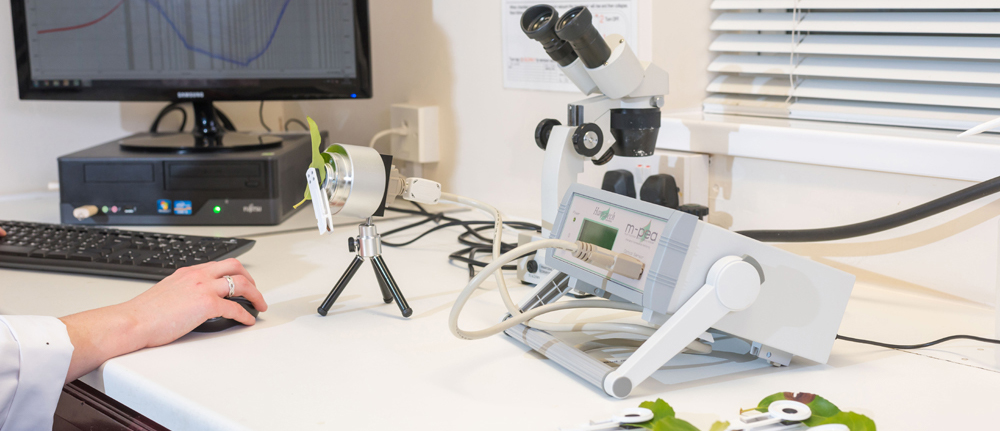
縮寫
| Absorption flux | 吸收通量 | | Chlorophyll | 葉綠素 | | Chlorophyll fluorescence | 葉綠素?zé)晒?/span> | | Cross section of the sample | 樣品橫截面 | | Cytochrome b6f | 細(xì)胞色素b6f | | Delayed (chlorophyll) fluorescence | 延遲(葉綠素)熒光 | | Drought factor index | 干旱因子指數(shù) | | Light-harvesting complex (of PSII) | PSII捕光色素復(fù)合體 | | Oxygen-evolving complex | 放氧復(fù)合體 | | Excited PSII reaction center | 激發(fā)的PSII反應(yīng)中心 | | PSI reaction center | PSI反應(yīng)中心 | | Photosynthetically active radiation | 光合有效輻射 | | Plastocyanin | 質(zhì)體藍(lán)素 | | Principal component analysis | 主成分分析 | | Prompt (chlorophyll) fluorescence | 瞬時(葉綠素)熒光 | | Pheophytin | 去鎂葉綠素 | | Plastoquinone | 質(zhì)體醌 | | Photosystem I, II | 光系統(tǒng)I, II | | Primary plastoquinone electron acceptor of PSII | PSII初級質(zhì)體醌電子受體 | | Secondary plastoquinone electron acceptor | 次級質(zhì)體醌電子受體 | | Reaction center | 反應(yīng)中心 | | Reactive oxygen species | 活性氧 | 在21世紀(jì),全球農(nóng)業(yè)必須生產(chǎn)更多的糧食來維持不斷增長的人口(Beddington et al. 2012)。然而,這一目標(biāo)受到人為氣候變化的威脅,這種變化有可能顯著降低受影響地區(qū)的糧食產(chǎn)量(Lobell et al.2008)。最近的研究表明,葉綠素?zé)晒?/span>(ChlF)測量可以為改進(jìn)全球農(nóng)業(yè)生產(chǎn)力模型提供獨特的基準(zhǔn),提高氣候變化情景下作物產(chǎn)量預(yù)測的可靠性(Guanter et al. 2014; Malaspina et al. 2014)。更廣泛地說,ChlF技術(shù)正在成為農(nóng)業(yè)、環(huán)境和生態(tài)研究中的一個非常強大的工具(Gottardiniet al. 2014)。它的一個主要優(yōu)點是ChlF技術(shù)是一種非侵入性的工具,允許科學(xué)家在不破壞被測樣品的情況下獲得光合過程的豐富信息。在自然條件下,植物受到許多不利的環(huán)境脅迫因子的影響。這些會破壞光合器官,導(dǎo)致植物生產(chǎn)力和總產(chǎn)量下降。光合作用對環(huán)境脅迫特別敏感(Kalaji et al. 2012),使光合測量成為植物脅迫研究的重要組成部分。然而,傳統(tǒng)的方法,甚至是技術(shù)上先進(jìn)的方法,如通過氣體交換(CO2、H2O和O2)測量光合速率,需要耗費大量時間和人力,且提供的有關(guān)整體光合功能的信息并不完整。相比之下,ChlF測量是一種簡單、無損、廉價和快速的工具,可用于分析光依賴性光合反應(yīng)和間接評估同一樣本組織中的葉綠素含量(Govindjee 1995; Papageorgiou & Govindjee 2011; Stirbet & Govindjee 2011, 2012)。ChlF方法的這些技術(shù)優(yōu)勢使其成為植物育種家(例如作物表型和監(jiān)測)、生物技術(shù)學(xué)家、植物生理學(xué)家、林業(yè)工作者、生態(tài)學(xué)家和環(huán)境學(xué)家的流行技術(shù)。
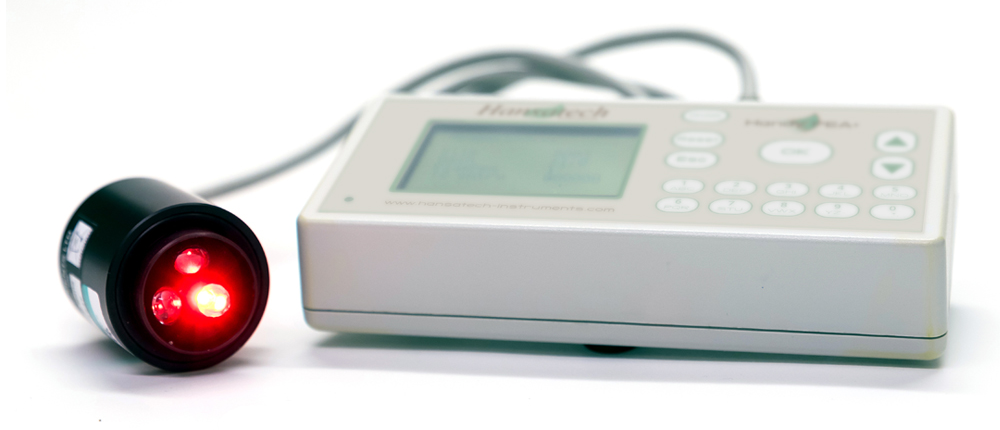
關(guān)鍵的是,從植物脅迫研究的角度來看,ChlF測量還提供了有關(guān)植物生理狀況的間接信息。通過分析葉綠素?zé)晒?/span>(ChlF)誘導(dǎo)曲線,可以評估光系統(tǒng)II(PSII)和光合電子傳遞鏈的生理狀況。它還提供了光依賴的光化學(xué)反應(yīng)和光無關(guān)的生化反應(yīng)的相關(guān)信息。總的來說,ChlF測量直接或間接地與依賴光的光合反應(yīng)的所有階段有關(guān),包括水的光解、電子傳遞、類囊體膜上pH梯度的形成、ATP合成以及光合機構(gòu)的一般生物能條件等(Bernát et al. 2012)。 許多ChlF技術(shù)和應(yīng)用現(xiàn)在已經(jīng)開發(fā)出來,為植物光合作用研究提供了豐富的技術(shù)手段。本文綜述了連續(xù)激發(fā)式熒光儀測量的快速葉綠素?zé)晒鈩恿W(xué)曲線(OJIP)的相關(guān)學(xué)術(shù)成果。這些研究是通過開發(fā)一個可靠的數(shù)學(xué)模型JIP-test(Strasser et al. 2004),允許分析在不到1s內(nèi)發(fā)生的熒光變化。此類分析提供了關(guān)于PSII反應(yīng)中心、天線以及PSII的供體和受體側(cè)的狀態(tài)和功能的詳細(xì)信息。綜述了脅迫因子對光化學(xué)過程的影響,對快速ChlF動力學(xué)和相關(guān)生物物理參數(shù)的變化規(guī)律。暗適應(yīng)葉片照光后可獲得多相葉綠素?zé)晒庹T導(dǎo)曲線(O–J–I–P-瞬變)(圖1)。曲線的軌跡提供了有關(guān)光合機構(gòu)結(jié)構(gòu)和功能的大量信息(Kautsky & Hirsch 1931; Schreiber et al. 1994)。
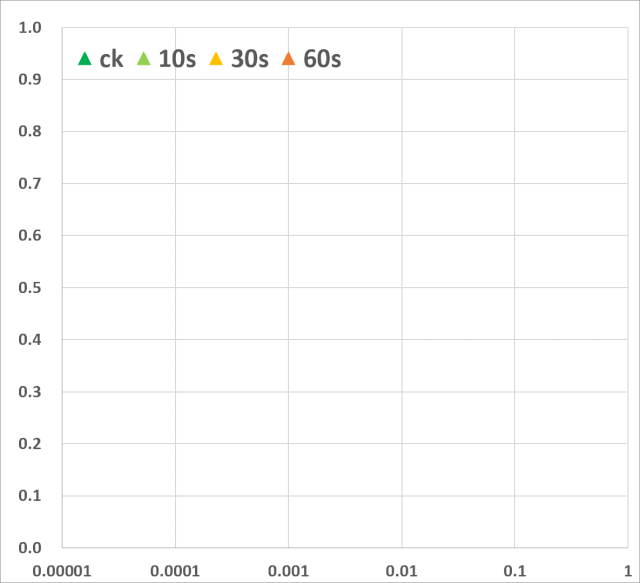
JIP-test是基于多相快速葉綠素?zé)晒獾纳仙A段,用于研究光依賴性反應(yīng)與ChlF的相關(guān)性。它基于類囊體膜的“能量流”理論(Strasser et al. 2000)。這個理論可以用簡單的代數(shù)方程來計算,代表每一個被檢測的捕光復(fù)合體的總能量流入和流出之間的平衡,并提供關(guān)于吸收能量的可能分配的信息。利用這些方程,可以描述PSII復(fù)合體之間的能量通信(也稱為“聚集grouping”或“連通性connectivity”和“總體分組概率overall grouping probability”)(Stirbet 2013)。 JIP-test(OJIP)的名稱來源于ChlF信號形成的感應(yīng)曲線上的特定位點(圖1):這些位點對應(yīng)于PSII原初電子受體(Pheo)和QA的逐漸還原。誘導(dǎo)曲線的形狀取決于PSII各組分間的聚集性(L-band)(Tsimilli-Michaeland Strasser 2013)和電子供體OEC→P680+以及QA-電子的接收之間的平衡(K-band)(Strasser et al. 2005)。 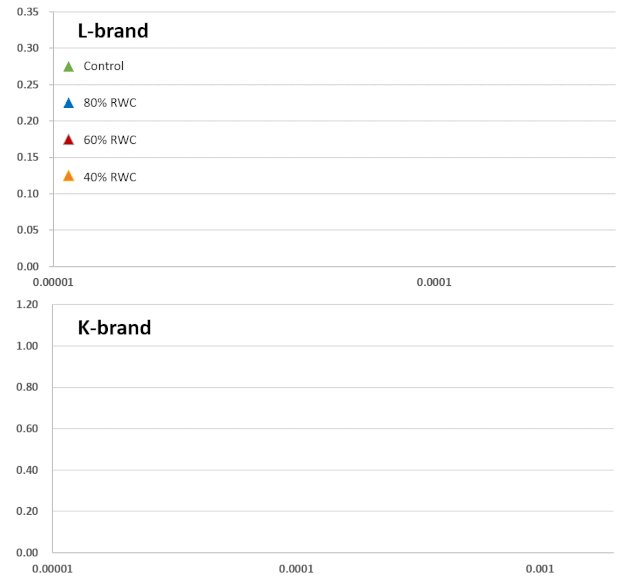
O~J相的熒光上升階段與部分PSII反應(yīng)中心的閉合相關(guān),反應(yīng)了QA的還原水平,其還原程度取決于捕獲速率以及QA被QB和其余電子傳遞鏈成員氧化的速率。 誘導(dǎo)曲線的J~I相與次級電子受體QB、PQ、Cyt b6f和PC的還原程度相關(guān)。誘導(dǎo)曲線的I~P相的上升通常歸因于PSI受體側(cè)電子受體(鐵氧還原蛋白、中間受體和NADP)的還原。 高溫、強光、缺氮或干旱脅迫會抑制放氧復(fù)合體OEC并阻礙OEC與酪氨酸之間的電子傳遞(Guha et al. 2013)。脅迫條件下,在ChlF誘導(dǎo)曲線200~300μs范圍內(nèi)會出現(xiàn)一個波峰——K-band,表明OEC已受到破壞。
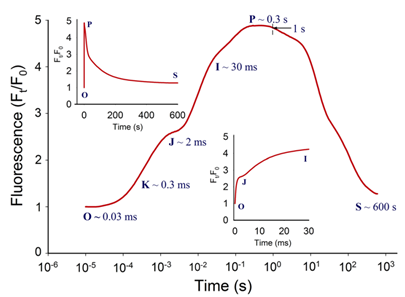
圖1:典型的植物葉綠素?zé)晒舛嘞鄤恿W(xué)曲線(主圖),曲線以對數(shù)時間刻度(10μs~600s)繪制。左上部插圖顯示了按常規(guī)時間標(biāo)度繪制的相同曲線。右下方插圖按常規(guī)時間標(biāo)度繪制的OJIP瞬態(tài)(0-30ms)的初始部分。時間標(biāo)記是指JIP-test用于計算結(jié)構(gòu)和功能參數(shù)的選定時間點。 表征PAR能量吸收和電子傳遞的JIP-test參數(shù)可主要分為以下四組:(1)基本測量值和計算值[熒光(Ft)、可變熒光(Vt)值和初始斜率等];(2)量子產(chǎn)率和概率;(3)能量通量;和(4)性能指數(shù)。表征能量通量的生物物理參數(shù)分為specific和phenomenological兩大類。specific參數(shù)按反應(yīng)中心(RC)計算,phenomenological參數(shù)按樣品截面(CS)計算。性能指數(shù)(PI)由幾個獨立參數(shù)的乘積計算得出,分別包括反應(yīng)中心的密度、初級光化學(xué)反應(yīng)的量子效率和電子傳遞中激發(fā)能的轉(zhuǎn)換(Strasser et al. 2000, 2004, 2010; Zushi et al. 2012)。性能指數(shù)被創(chuàng)建為非特定參數(shù),主要用于實際應(yīng)用,如篩選在田間條件下增強的應(yīng)力耐受性(Srivastava et al. 1999; Strasser et al. 2004; Brestic & Zivcak 2013)。葉綠素?zé)晒鈩恿W(xué)也可以用來揭示光合機構(gòu)的PSII異質(zhì)性。PSII在天線色素和還原側(cè)方面具有天然異質(zhì)性。天線異質(zhì)性包括天線尺寸和天線色素分子組分間的連通性(或聚集性)差異。PSII反應(yīng)中心基于天線尺寸可分為三類:alpha (α), beta (β)和gamma (γ) (Melis & Homann 1976),其主要區(qū)別在于天線壽命和伴生葉綠素的數(shù)量。還原側(cè)的異質(zhì)性主要與從QA開始電子傳遞的能力有關(guān)。可將電子由QA傳遞給QB的反應(yīng)中心命名為可還原QB的反應(yīng)中心(QB reducing centers),而不具備此能力的反應(yīng)中心稱之為不可還原QB的反應(yīng)中心(QB non-reducing centers)。Jajoo(2013)回顧了PSII異質(zhì)性的具體特征。近期研究表明,高溫(Mathur et al. 2011b),高鹽(Mehta et al. 2010a)以及如多環(huán)芳烴(PAH)等污染物(Tomarand Jajoo 2013, 2014)脅迫下均會引起PSII異質(zhì)性的變化。PSII異質(zhì)性的變化可能與活躍/不活躍反應(yīng)中心的數(shù)量有關(guān),在各種脅迫條件下活躍的α反應(yīng)中心轉(zhuǎn)換為非活躍的β和γ反應(yīng)中心,同時不可還原QB的反應(yīng)中心數(shù)量也隨之增多。葉綠素?zé)晒鈩恿W(xué)參數(shù)對不同非生物脅迫的響應(yīng)在下面的章節(jié)中,我們回顧了ChlF動力學(xué)可以作為氣候變化和人類活動(如高溫和低溫、干旱、鹽分、營養(yǎng)缺乏和重金屬)負(fù)面影響的有效指標(biāo)的證據(jù)。 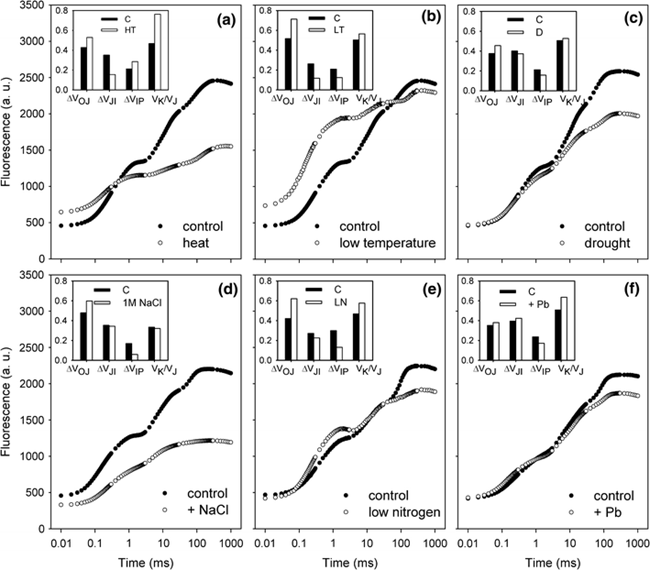
圖2:不同脅迫條件下小麥(Triticum sp.L.)葉綠素?zé)晒獾腛(K)JIP瞬態(tài)與非脅迫下的比較。插入顯示了O-J相(∆VOJ)、J-I相(∆VJI)、I-P相(∆VIP)的相對可變熒光振幅的變化,以及0.3 ms可變熒光(VK/VJ)與2ms可變熒光比值(VJ)的變化,作為PSII供體側(cè)限制(K-band)的指標(biāo)。各圖顯示了相對于非脅迫狀態(tài)下植物(control,C)的瞬時熒光曲線:a熱脅迫(高溫脅迫8h,中度光化光照射,葉片溫度約40℃);b低溫脅迫10d(10/6℃:日間/夜間);c重度干旱脅迫(停止灌溉后12d,葉片含水量約60%);d鹽脅迫(NaCl);e氮缺乏脅迫(低氮,LN);f鉛脅迫。 氣候變化可能會增加植物的熱脅迫,限制生產(chǎn)力和生物量的積累。光合作用是植物細(xì)胞過程中對高溫最敏感的過程(Sharkey & Schrader 2006),高溫會導(dǎo)致PSII電子受體還原-氧化特性發(fā)生變化,并降低兩個光系統(tǒng)中光合電子傳輸?shù)男?/span>(Mathur et al. 2014)。熱脅迫會影響ChlF參數(shù)的值(圖2a)。例如蘋果Malus x domestica Borkh在高溫脅迫下,其QA-/RC和QB-/QA-的比率均產(chǎn)生下降,同時PSII最大量子產(chǎn)率(Fv/Fm)降低而最小熒光Fo升高(Chen et al. 2009; Brestic et al. 2013)。高溫脅迫同樣會對O-J-I-P曲線的形狀產(chǎn)生影響,會導(dǎo)致Fm的降低和Fo升高。Fo的升高可能是由于捕光色素復(fù)合體LHC II從PSII復(fù)合體上解離、PSII光化學(xué)反應(yīng)的失活或還原的電子受體QA至QB電子流傳遞受到抑制而導(dǎo)致的(Mathur et al. 2011a)。例如,由菠菜和水稻中觀察到的Fo升高歸因于LHC II與PSII復(fù)合體的不可逆解離和PSII的部分可逆性失活(Yamane et al. 1997)。Fm的降低可能與葉綠素蛋白的變性有關(guān)(Yamane et al. 1997)。K峰(300μs)是熱應(yīng)激一個極佳的指示指標(biāo),可用于指示放氧復(fù)合體OEC的解離和去鎂葉綠素Pheo與初級電子受體QA間的電子傳遞情況(Strasser et al. 2000; Lazár 2006)。在小麥中,35℃處理時對凈光合速率未產(chǎn)生影響,而當(dāng)45℃處理時則對OEC產(chǎn)生了不可逆的損傷(Schreiber et al. 2012)。K峰出現(xiàn)的直接原因是電子由P680向PSII電子受體的流出量遠(yuǎn)超于電子由PSII供體側(cè)向P680的流入量。同時K峰也會受到光系統(tǒng)II之間能量關(guān)系變化的影響。FK/FJ比率的增大表明熱脅迫抑制了OEC的電子供應(yīng)(Srivastava & Strasser 1995)。快速ChlF技術(shù)也是監(jiān)測PSII熱穩(wěn)定性有效方法。最有效的方法是評估臨界溫度,即在臨界溫度以上觀測相應(yīng)參數(shù)的快速增加或減少(Brestic & Zivcak 2013)。在作物育種中,一些基因型可以作為增強耐熱性的供體。以熱處理菜豆(Phaseolus vulgaris L.)品系為例,通過ChlF誘導(dǎo)的變化來監(jiān)測其脅迫反應(yīng)和恢復(fù)狀況,并應(yīng)用JIP-test進(jìn)行分析(Stefanov et al. 2011)。Brestic et al. (2012)利用快速ChlF動力學(xué)方法對30個不同地理來源冬小麥基因型的PSII熱穩(wěn)定性進(jìn)行了研究。Gautum et al. (2014)證明ChlF法在硬粒小麥基因型篩選中比常規(guī)方法(如收獲指數(shù)、籽粒灌漿等)更有效。在某些緯度地區(qū),低溫是限制作物產(chǎn)量的主要因素(Yang et al. 2009)。在北半球冬季和早春的低溫通常伴隨著強光照現(xiàn)象,在這種環(huán)境條件下,會導(dǎo)致植物類囊體結(jié)構(gòu)退化和光依賴性的光合反應(yīng)的扭曲(Suzuki et al. 2011)。冷脅迫同樣會影響ChlF參數(shù)(圖2b)。例如,低溫脅迫下苦瓜(Momordica charantia L.)植株的葉綠素含量、PSII供體側(cè)OEC效率、光化學(xué)猝滅和開放的PSII反應(yīng)中心效率均降低(Yang et al. 2009)。某些具有極佳低溫耐受性的物種表現(xiàn)出較少的PSII光抑制。例如,低溫脅迫下豌豆植株的ChlF參數(shù)只有極小的變化(Strauss et al. 2006; Strebet al. 2008)。干旱脅迫對光合器官的影響是眾所周知的。在中等干旱強度下它們通常開始主要是氣孔效應(yīng),嚴(yán)重或長期干旱脅迫最終會導(dǎo)致代謝和結(jié)構(gòu)性變化(Jedmowski et al. 2013)。這種最終的變化也與光保護(hù)和抗氧化功能和途徑的增強有關(guān)(Chaves et al. 2009)。與PSI相比,PSII具有較高的抗缺水能力,因此只有在極端干旱的情況下才會產(chǎn)生負(fù)面影響(Lauriano et al. 2006)。ChlF測量表明,在干旱條件下,通過調(diào)節(jié)光系統(tǒng)之間的能量分布和激活交替電子流,增強了PSII和PSI光化學(xué)的保護(hù)作用(Zivcak et al. 2013)。干旱脅迫可增強PSII對熱脅迫的抗性,表現(xiàn)為OJIP瞬態(tài)的K峰的消失(見圖2c,Oukarroum et al. 2012)。ChlF方法可用于篩選耐旱性基因型(Guha et al. 2013)。OJIP熒光曲線上升的最初2~3ms階段與初級光化學(xué)反應(yīng)相關(guān),Oukarroum et al. (2007)建議脅迫激發(fā)出現(xiàn)的L-band和K-band可作為評估應(yīng)對和恢復(fù)干旱脅迫潛力的有效工具。L-band受PSII各組分間激發(fā)能傳遞的影響,通常表示為連通性(connectivity)或聚集性(grouping)(Strasser & Stirbet 1998)。不論是突變(Brestic et al. 2014)或環(huán)境條件(Zivcak et al. 2014a)而引發(fā)的PSII天線色素組分的改變,同樣會使L-band受到影響。K-band的出現(xiàn)與放氧復(fù)合體OEC的解離有關(guān)(Guisse et al. 1995)。因此,O-L-K-J-I-P熒光瞬態(tài)的測量和利用JIP-test進(jìn)行的分析可以作為干旱脅迫耐性和干旱脅迫可見癥狀出現(xiàn)前生理紊亂的有效指示工具。性能指數(shù)(PI)是應(yīng)用最廣泛的ChlF OJIP曲線參數(shù),為我們提供了關(guān)于植物狀態(tài)和活力的定量信息。PI由三個獨立特性的參數(shù)乘積組成:每個葉綠素分反應(yīng)中心濃度、初級光化學(xué)反應(yīng)相關(guān)參數(shù)和電子傳遞相關(guān)參數(shù)(Strasser et al. 2004)。因此PI對天線特性、捕獲效率或除QA外的電子傳遞的變化都很敏感。例如,冬小麥在花后長期干旱脅迫下,PI值降低。此外,根據(jù)干旱脅迫下的PI值估算的小麥基因型的耐旱性與以產(chǎn)量為指標(biāo)的抗旱性也有很好的相關(guān)性(Zivcaket al. 2008)。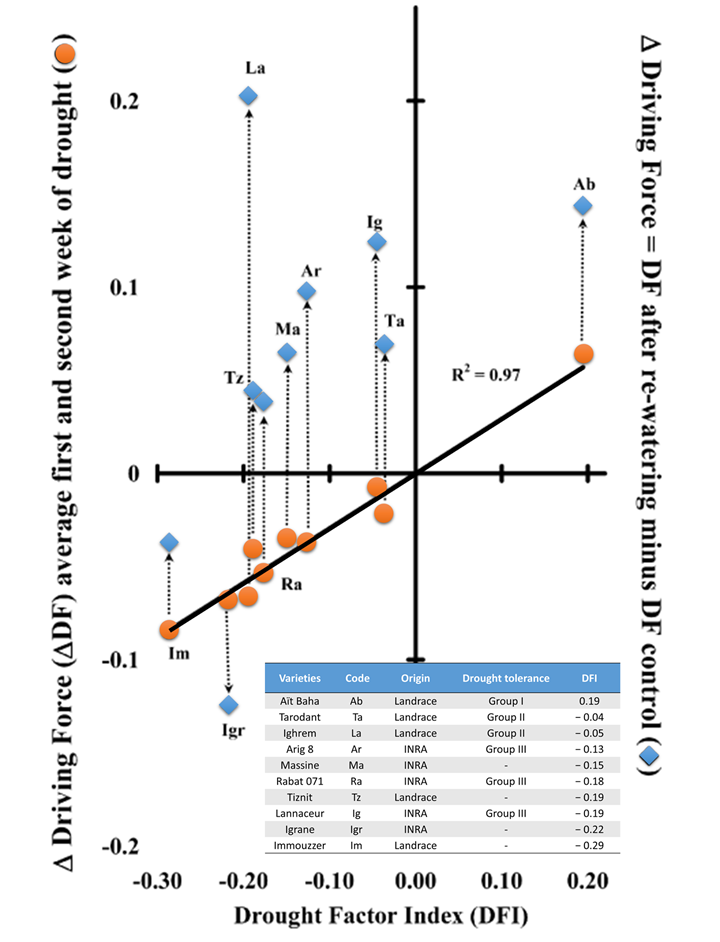 PI與干旱因子指數(shù)(DFI)密切相關(guān),DFI表示在一定的干旱脅迫時間內(nèi),PI的相對減少量。Strauss等人使用了DFI方法(Strauss et al. 2006)評估不同大豆基因型的耐暗冷性。DFI還被用于對10個大麥品種(Oukarroum et al. 2007)和21種芝麻突變體種質(zhì)資源(Boureima et al. 2012)的耐旱性進(jìn)行排序。利用PI參數(shù)和ChlF快速誘導(dǎo)曲線成功篩選了來自埃及的**耐性和最敏感的大麥和高粱品種(Jedmowskiet al. 2013)。這些研究表明,在PSII水平上可以區(qū)分耐旱性和敏感性品種。在干旱脅迫下同樣觀察到ABS/RC的增大(Van Heerden et al. 2007; Gomeset al. 2012),這可能是由于部分PSIIRCs失活或天線尺寸增大而導(dǎo)致的。干旱脅迫也會影響OJIP曲線I~P相的相對振幅。I~P相是熒光曲線上升的最慢階段(約30~200ms),與質(zhì)體籃素PC和PSI中P700+的再還原有關(guān)(Schreiber et al. 1989; Schansker et al. 2003)。I~P相似乎與PSI反應(yīng)中心的含量(Ceppiet al. 2012)或由820nm透射測量得到的線性電子傳遞活性(Zivcak et al. 2014a)有關(guān)。例如,不同大麥品種的I~P損失程度取決于它們的耐旱性(Oukarroum et al. 2009; Ceppi et al. 2012)。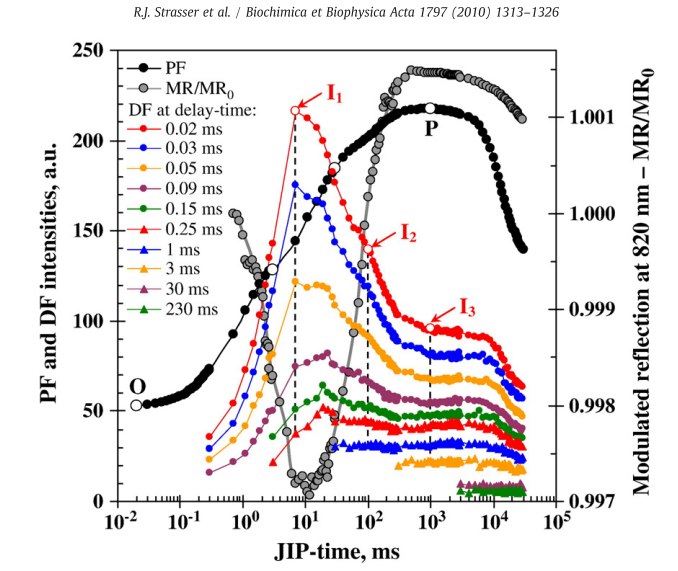 ChlF是在光合樣品在暗到光轉(zhuǎn)換之后發(fā)射的,而延遲熒光(DF)的發(fā)射發(fā)生在光到暗的轉(zhuǎn)換過程中(Goltsev et al. 2009; Strasser et al. 2010; Kalaji et al. 2012)。DF被認(rèn)為反映了還原的初級電子受體QA-和光誘導(dǎo)電荷分離形成的PSII氧化供體P680+的在黑暗狀態(tài)下的再復(fù)合。DF誘導(dǎo)曲線的形狀取決于樣品材料類型和其生理狀態(tài)。使用Hansatech公司M-PEA多功能植物效率分析儀同時測量ChlF 瞬時熒光OJIP曲線和DF曲線,目前被用于獲取不同光合反映的速率常數(shù)(Strasser et al. 2010)。使用此技術(shù)Goltsev et al. (2012)觀察到在干旱脅迫下QA-的再氧化受到抑制,PSII反應(yīng)中心光誘導(dǎo)電子傳遞量子產(chǎn)率被抑制,調(diào)制反射信號(820nm)的光誘導(dǎo)動力學(xué)快速相降低。 植物對鹽脅迫的反應(yīng)是由多個方面決定的,如特定基因的表達(dá)、植物的發(fā)育階段、甜菜堿的積累,這些甜菜堿通過穩(wěn)定PSII復(fù)合體的外部蛋白質(zhì)來保護(hù)光合機構(gòu)(Murata et al. 1992)。鹽分脅迫干擾了從RCs至質(zhì)體醌庫的電子傳遞(Strasser et al. 2000; 圖2d)。Schreiber et al. (1994)鑒定出OEC是光合電子傳遞鏈中最敏感的組分之一。OEC性能的下降通常是由于電子傳遞紊亂引起的。鹽脅迫下同樣可觀察到ChlF參數(shù)和PSII功能性的改變。在高鹽脅迫下,由于LHC II和PSII解離導(dǎo)致了PSII反映中心捕獲電子效率的下降(Havaux 1993)。在許多物種中,如大麥(Kalaji & Rutkowska 2004)、煙草Nicotiana tobacum L. (Yang et al. 2008)、甚至某些鹽生植物如草珊瑚Sarcocorniafruticosa L中,均觀察到了PSII最大量子效率(Fv/Fm)的降低和非光化學(xué)淬滅(NPQ或qN)的增加。此外,番茄和黃瓜幼苗在鹽分脅迫下,光下PSII光化學(xué)效率(ΦPSII)、電子傳遞效率(ETR)和光照下PSII開放反應(yīng)中心的效率均受影響而降低(He et al. 2009; Zhang & Sharkey 2009)。鹽脅迫對小麥的傷害主要表現(xiàn)在供體側(cè),而非受體側(cè),這種損傷在PSII受體側(cè)是完全可逆的(約100%),而供體側(cè)的恢復(fù)率小于85%(Mehta et al. 2010b)。鹽脅迫的滲透和離子效應(yīng)也通過ChlF測量得到了有效區(qū)分(Singh-Tomar et al. 2012)。特定營養(yǎng)元素(N、P、K、Ca、Mg、S或Fe)的缺乏會破壞光合器官的功能,降低PSII光化學(xué)效率并改變ChlF參數(shù)的值(Smethurst et al. 2005)。氮素(N)缺乏是限制植物生長的關(guān)鍵因素,是所有蛋白質(zhì)、核酸和其他有機化合物的組成部分。氮素缺乏導(dǎo)致類囊體膜的改變并擾亂其功能(圖2e),并進(jìn)一步加速葉綠體衰老和質(zhì)體小球的形成(Wu et al. 2006)。氮也是RuBisCO光合復(fù)合物、卡爾文循環(huán)酶、葉綠素和類胡蘿卜素中的重要元素(Correia et al. 2005)。氮缺乏會導(dǎo)致蒸騰作用、氣孔導(dǎo)度、葉綠素和類胡蘿卜素含量以及可溶性糖濃度的降低(Huang et al. 2004)。氮攝取不足也會降低PSII中的電子受體庫,降低RuBisCO和磷酸烯醇式丙酮酸羧化酶(PEPCase)的活性(Correia et al. 2005)。JIP-test分析已經(jīng)在處理氮缺乏的研究中多次應(yīng)用,并且已經(jīng)很好地描述了氮供應(yīng)不足對PSII的影響(Redillas et al. 2011, Li et al. 2012)。特別是,氮素缺乏導(dǎo)致的反應(yīng)中心密度顯著降低(Dudeja & Chaudhary 2005)。同時,高氮處理對大豆(Van Heerden et al. 2004)、玉米(Li et al. 2012)和小麥(Zivcak et Val. 2014b)中PI值的積極影響已經(jīng)得到了證明。磷對植物的生長發(fā)育也是必不可少的。磷缺乏將主要導(dǎo)致籽粒和類囊體膜結(jié)構(gòu)改變、捕光復(fù)合體吸收PAR的下降,從而導(dǎo)致PSII活性的降低(Foyer & Spencer 1986)。磷缺乏還對NADPH的再生過程產(chǎn)生不利影響,降低光合作用的量子產(chǎn)率、羧化效率和電子傳遞效率(Wu et al. 2006)。JIP-test已成功應(yīng)用于磷缺乏脅迫下植物PSII活性或效率的評估(Kruger et al. 1997; Tsimilli-Michael & Strasser 2008)。此外各種研究證明,JIP-test參數(shù)和氣體交換或植物生長參數(shù)具有高度相關(guān)性(Strasser et al. 2000)。鉀(K)在細(xì)胞滲透調(diào)節(jié)中起著關(guān)鍵作用:鉀離子是保持類囊體膜上的pH梯度所必需的(Rampino et al. 2006)。鉀缺乏會導(dǎo)致氣孔導(dǎo)度阻力增加,限制二氧化碳通過氣孔的擴散。在光合作用中,鉀在許多酶的激活和ATP合成中的重要作用可能比它在調(diào)控氣孔功能中的作用重要的多。然而鉀缺乏對光合組織效率和PSII功能的影響知之甚少。然而,在缺鉀條件下,一些光合參數(shù)如電子傳遞效率(ETR)和最大量子產(chǎn)率(Fv/Fm)都會降低(Schweiger et al. 1996)。有許多研究使用快速ChlF參數(shù)來分析其他礦物質(zhì)缺乏對光化學(xué)功能的影響,例如鈣(Liu et al. 2009; Lauriano et al. 2006)、鎂(Smethurst et al. 2005)和鐵(Molassiotis et al. 2006)。由于許多營養(yǎng)物質(zhì)對PSII光化學(xué)反應(yīng)有特殊的影響,這里的問題是是否有可能利用葉綠素?zé)晒鈩恿W(xué)來識別營養(yǎng)缺乏。盡管這一問題仍然懸而未決,Kalaji et al. (2014a, b)能夠利用JIP-test參數(shù)的主成分分析(PCA)來識別番茄和玉米的主要營養(yǎng)素的缺乏情況。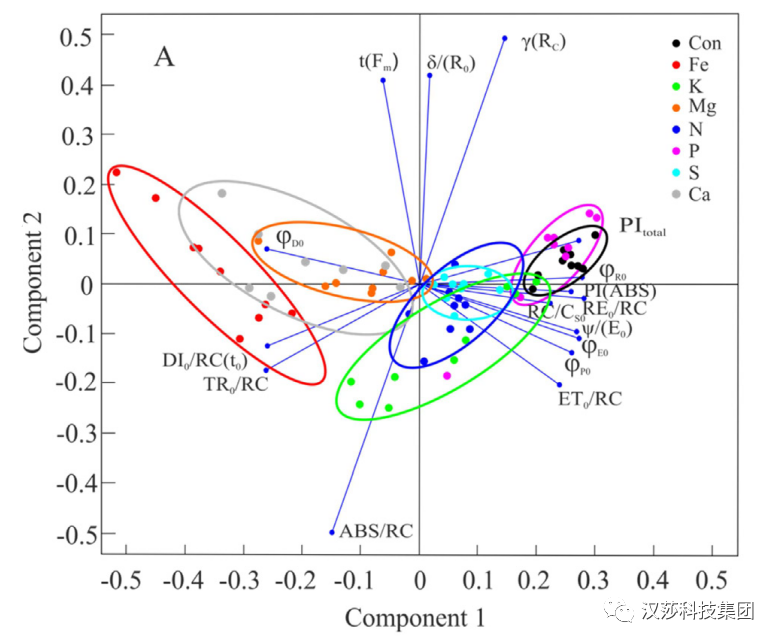 高濃度的重金屬脅迫會破壞光合作用進(jìn)程,但特定重金屬離子的影響可能是物種特異性的(Antosiewicz 2005; Mishra & Dubey 2005)。PSI被認(rèn)為比PSII更能耐受重金屬的脅迫(Romanowska et al. 2006; Tuba et al. 2010)。鎘(Cd)是毒性最大的重金屬之一,可在生物體內(nèi)富集。環(huán)境中鎘的來源包括磷肥和工業(yè)廢料(Romanowska et al. 2006; Kalaji & Łoboda 2007)。然而,鎘似乎不會影響光合色素的含量。對油菜幼苗的研究表明,在Cd存在下生長2周后,葉綠素a、葉綠素b和類胡蘿卜素的含量沒有顯著變化(Janeczko et al. 2005)。然而,Cd確實對光合過程的光化學(xué)效率有負(fù)面影響。PSII比PSI對鎘的影響更敏感,表明Cd以更大的強度破壞PSII功能(Mallick & Mohn 2003)。Cd同時影響PSII的供體和受體側(cè)。在供體側(cè),它抑制OEC,而在受體側(cè),由于LHCII復(fù)合體的解離導(dǎo)致電子傳遞紊亂,抑制了QA-和QB-之間的電子傳遞(Sigfridsson et al. 2004)。Cd脅迫同樣會引發(fā)非光學(xué)淬滅或熱耗散的升高(Janeczko et al. 2005)。對油菜JIP-test參數(shù)的分析表明,Cd引起了油菜葉片橫截面比能流如RC/CS、ETo/CS和OEC活性的降低(Janeczko et al. 2005)。PSII最大量子效率Fv/Fm是Cd脅迫影響最不敏感的參數(shù)。植物對鎘的抗性與“清除”活性氧的能力、激活抗氧化酶[特別是過氧化物酶(Ekmekci et al. 2008)]以及合成抗氧化化合物[如谷胱甘肽(Streb et al. 2008)]等保護(hù)機制的啟動有關(guān)。鉛對植物也有有害影響。土壤和植物中鉛的主要源頭來自于燃煤發(fā)電廠、汽車尾氣和工業(yè)廢棄物(Mishra & Dubey 2005)。鉛會引起呼吸代謝的改變,導(dǎo)致線粒體產(chǎn)生高能化合物,使ATP含量和ATP/ADP比值升高(Romanowska et al. 2002)。鉛脅迫下植物光合作用效率的降低是由于葉綠體超微結(jié)構(gòu)和類囊體膜脂成分的破壞,以及葉綠素和類胡蘿卜素合成的減少造成的(Sharma & Dubey 2005)。鉛脅迫會阻斷如鎂和鐵等營養(yǎng)元素的吸收,而鎂和鐵是光合作用所必需的。此外鉛還會導(dǎo)致OEC復(fù)合體的解離,并將Ca,Cl,Mn化合物從OEC復(fù)合體中分離去除(Sharma & Dubey 2005; Romanowska et al. 2006)。與對照組相比,暴露于鉛脅迫的植物的O–J–I–P誘導(dǎo)曲線中I和P階的熒光強度降低(圖2f),并出現(xiàn)K峰(Kalaji & Łoboda 2007)。ChlF誘導(dǎo)曲線上出現(xiàn)以上變化可能與OEC和PSII反應(yīng)中心之間的電子傳遞抑制有關(guān)(Strasser et al. 2004; Wu et al. 2008)。鉛脅迫模型表明,PSII內(nèi)的能量吸收和耗散很高,而電子捕獲和電子傳遞則大幅減少(Lazár & Jablonsky 2009)。用于快速ChlF動力學(xué)分析的數(shù)學(xué)模型,如JIP-test,是專門用來評估微秒級或毫秒級葉綠體氧化還原反應(yīng)級聯(lián)反應(yīng)的生物物理工具。盡管如此,早期的研究即已經(jīng)獲取了很多關(guān)于葉片生理狀態(tài)和熒光瞬態(tài)曲線形狀之間關(guān)系的有趣的經(jīng)驗理論(Strasser et al. 2000)。隨后又有許多文獻(xiàn)報道了葉片生理狀態(tài)與ChlF瞬變之間的直接關(guān)系。而常被忽略的一個事實是測量得到的信號是一個復(fù)合信號(見上文關(guān)于PSII異質(zhì)性的討論),而該信號是與樣品測定時的生理狀態(tài)和環(huán)境條件高度相關(guān)的。因此,需要我們多方考慮各種因素的綜合作用,以避免錯誤地或過度簡化的得出結(jié)論(Evans 2009)。快速葉綠素?zé)晒饧夹g(shù)操作簡單、快速,但如對其基本理論原理了解不深,很容易造成對該技術(shù)的不當(dāng)應(yīng)用。綜合參數(shù)的使用,如性能指數(shù)(PI)可能比復(fù)雜的特定生物物理參數(shù)更有用,后者需要對光化學(xué)過程有更深入的理解才能正確地解釋數(shù)據(jù)。Stirbet & Govindjee (2011)深入探討了JIP-test在分析OJIP曲線中的各方利弊。為了避免ChlF應(yīng)用中的錯誤,強烈建議所有用戶熟悉該技術(shù)的各種理論細(xì)節(jié)(見Kalaji et al. 2014a綜述文章)。本文介紹了葉綠素?zé)晒饧夹g(shù)在植物科學(xué)、農(nóng)業(yè)和生態(tài)研究中應(yīng)用的**信息。葉綠素?zé)晒獾臏y量信號及其統(tǒng)計分析(如JIP-test)可用于預(yù)測、監(jiān)測和識別植物的脅迫。因此,它可以作為一種生物指示劑應(yīng)用于幾乎所有的植物生態(tài)學(xué)研究中。ChlF測量的多功能性意味著它們可以在單一植物的水平上應(yīng)用于草原、農(nóng)田甚至海洋生態(tài)系統(tǒng)。然而,這種潛在的多功能性強調(diào)了需要進(jìn)行更實際和概念性的研究,使科學(xué)家能夠獲得有關(guān)植物生長和健康的可靠信息。這樣一種方法不僅將使我們對光合作用的生理基礎(chǔ)的理解得到改善,而且還將有助于了解和補救氣候變化對作物產(chǎn)量和糧食安全的影響。
- AntosiewiczDM (2005) Study of calcium-dependent lead-tolerance on plants differing intheir level of Ca-deficiency tolerance. Environ Pollut 134(1):23–34
- BeddingtonJ, Asaduzzaman M, Clark M, Bremauntz AF, Guillou M, Howlett D, Jahn M, Lin E,Mamo T, Negra C (2012) What next for agriculture after Durban? Science335:289–290
- BernátG, Schreiber U, Sendtko E, Stadnichuk IN, Rexroth S, Rögner M, Koenig F (2012)Unique properties vs. common themes: the atypical cyanobacterium Gloeobacterviolaceus PCC 7421 is capable of state transitions and blue-light-inducedfluorescence quenching. Plant Cell Physiol 53(3):528–542
- BoureimaS, Oukarroum A, Diouf M, Cisse N, Van Damme P (2012) Screening for droughttolerance in mutant germplasm of sesame (Sesamum indicum) probing by chlorophylla fluorescence. Environ Exp Bot 81:37–43
- BresticM, Zivcak M (2013) PSII Fluorescence techniques for measurement of drought andhigh temperature stress signal in crop plants: protocols and applications.Molecular stress physiology of plants. Springer, Berlin, pp 87–131
- BresticM, Zivcak M, Kalaji MH, Carpentier R, Allakhverdiev SI(2012) Photosystem IIthermostability in situ: environmentally induced acclimation andgenotype-specific reactions in Triticum aestivum L. Plant PhysiolBiochem 57:93–105
- BresticM, Zivcak M, Olsovska K, Repkova J (2013) Involvement of chlorophyll afluorescence analyses for identification of sensitiveness of the photosyntheticapparatus to high temperature in selected wheat genotypes. Photosynthesisresearch for food, fuel and the future. Springer, Berlin, pp 510–513
- BresticM, Zivcak M, Olsovska K, Shao HB, Kalaji MH, Allakhverdiev SI (2014) Reducedglutamine synthetase activity plays a role in control of photosyntheticresponses to high light in barley leaves. Plant Physiol Biochem 81:74–83.doi:10.1016/j.plaphy.2014.01.002
- CeppiMG, Oukarroum A, C ¸i c ¸ek N, Strasser RJ, Schansker G (2012) The IP amplitudeof the fluorescence rise OJIP is sensitive to changes in the photosystem Icontent of leaves: a study on plants exposed to magnesium and sulfatedeficiencies, drought stress and salt stress. Physiol Plant 144(3):277–288
- ChavesMM, Flexas J, Pinheiro C (2009) Photosynthesis under drought and salt stress:regulation mechanisms from whole plant to cell. Ann Bot 103(4):551–560
- ChenLS, Li P, Cheng L (2009) Comparison of thermotolerance of sun-exposed peel andshaded peel of ‘Fuji’apple. Environ Exp Bot 66(1):110–116
- CorreiaCM, Pereira JMM, Coutinho JF, Björn LO, Torres-Pereira JMG (2005) Ultraviolet-Bradiation and nitrogen affect the photosynthesis of maize: a Mediterraneanfield study. Eur J Agron 22(3):337–347
- DudejaSS, Chaudhary P (2005) Fast chlorophyll fluorescence transient and nitrogenfixing ability of chickpea nodulation variants. Photosynthetica 43:253–259
- Ekmekc¸i Y, Tanyolac D, Ayhan B (2008) Effects of cadmium on antioxidant enzyme andphotosynthetic activities in leaves of two maize cultivars. J Plant Physiol165(6):600–611
- EvansJR (2009) Potential errors in electron transport rates calculated fromchlorophyll fluorescence as revealed by a multilayer leaf model. Plant CellPhysiology 50(4):698–706
- FoyerC, Spencer C (1986) The relationship between phosphate status andphotosynthesis in leaves. Planta 167(3):369–375
- GautumA, Agrawal D, SaiPrasad SV, Jajoo A (2014) A quick method to screen high andlow yielding wheat cultivars exposed to high temperature. Physiol Mol BiolPlants. doi:10.1007/s12298-014-0252-4
- GoltsevV, Zaharieva I, Chernev P, Kouzmanova M, Kalaji MH, Yordanov I, Krasteva V,Alexandrov V, Stefanov D, Allakh-verdiev SI, Strasser RJ (2012) Drought-inducedmodifications of photosynthetic electron transport in intact leaves: analysisand use of neural networks as a tool for a rapid non-invasive estimation.Biochim Biophys Acta 1817:1490–1498
- GoltsevV, Zaharieva I, Chernev P, Strasser RJ (2009) Delayed chlorophyll fluorescenceas a monitor for physiological state of photosynthetic apparatus. BiotechnolBiotechnol Equip 23(Special Edition):452–457
- GomesMTG, da Luz AC, dos Santos MR, Batitucci MDCP, Silva DM, Falqueto AR (2012)Drought tolerance of passion fruit plants assessed by the OJIP chlorophyll afluorescence transient. Sci Hortic 142:49–56
- GottardiniE, Cristofori A, Cristofolini F, Nali C, Pellegrini E, Bussotti F, Ferretti M(2014) Chlorophyll-related indicators are linked to visible ozone symptoms:evidence from a field study on native Viburnum lantana L. plants innorthern Italy. Ecol Ind 39:65–74
- Govindjee(1995) Sixty-three years since Kautsky: chlorophyll a fluorescence. AusJ Plant Physiol 22:131–160
- GuanterL, Zhang Y, Jung M, Joiner J, Voigt M, Berry JA, Frankenberg C, Huete AR,Zarco-Tejada P, Lee JE (2014) Global and time-resolved monitoring of cropphotosynthesis with chlorophyll fluorescence. Proc Natl Acad Sci111(14):E1327–E1333
- GuhaA, Sengupta D, Reddy AR (2013) Polyphasic chlorophyll a fluorescencekinetics and leaf protein analyses to track dynamics of photosyntheticperformance in mulberry during progressive drought. J Photochem Photobiol B119:71–83
- GuisséB, Srivastava A, Strasser RJ (1995) Effects of high temperature and waterstress on the polyphasic chlorophyll a fluorescence transient of potatoleaves. In: Mathis P (ed) Photosynthesis: from light to biosphere. KluwerAcademic Publishers, Dordrecht, pp 913–916
- HavauxM (1993) Rapid photosynthetic adaptation to heat stress triggered in potatoleaves by moderately elevated temperatures. Plant Cell Environ 16(4):461–467
- HeY, Zhu Z, Yang J, Ni X, Zhu B (2009) Grafting increases the salt tolerance oftomato by improvement of photosynthesis and enhancement of antioxidant enzymesactivity. Environ Exp Bot 66(2):270–278
- HuangZA, Jiang DA, Yang Y, Sun JW, Jin SH (2004) Effects of nitrogen deficiency ongas exchange, chlorophyll fluorescence, and antioxidant enzymes in leaves ofrice plants. Photosynthetica 42(3):357–364
- JajooA (2013) Changes in photosystem II in response to salt stress. Ecophysiologyand responses of plants under salt stress. Springer, Berlin, pp 149–168
- JaneczkoA, Koscielniak J, Pilipowicz M, Szarek-Lukaszewska G, Skoczowski A (2005)Protection of winter rape photosystem 2 by 24-epibrassinolide under cadmiumstress. Photosynthetica 43(2):293–298
- JedmowskiC, Ashoub A, Bru ¨ggemann W (2013) Reactions of Egyptian landraces of Hordeumvulgare and Sorghum bicolor to drought stress, evaluated by the OJIP fluorescencetransient analysis. Acta Physiologiae Plantarum 35(2):345–354
- KalajiMH, Rutkowska A (2004) Reactions of photosynthetic apparatus of maize seedlingsto salt stress. Zesz Probl Post NaukRol 496:545–558
- KalajiMH, Carpentier R, Allakhverdiev SI, Bosa K (2012) Fluorescence parameters asearly indicators of light stress in barley. J Photochem Photobiol B 112:1–6
- KalajiMH, Schansker G, Ladle RJ, et al (2014a) Frequently asked questions about invivo chlorophyll fluorescence: practical issues. Photosynth Res. doi:10.1007/s11120-014-0024-6
- KalajiMH, Oukarroum A, Alexandrov V, Kouzmanova M, Brestic M, Zivcak M, Goltsev V(2014b) Identification of nutrient deficiency in maize and tomato plants by invivo chlorophyll a fluorescence measurements. Plant Physiol Biochem.doi:10.1016/j.plaphy.2014.03.029
- KautskyH, Hirsch A (1931) NeueVersuchezur Kohlensaureassimilation. Naturwissenschaften19:964
- KrugerGHJ, Tsimilli-Michael M, Strasser RJ (1997) Light stress provokes plastic andelastic modifications in structure and function of photosystem II in camellialeaves. Physiol Plant 101:265–277
- LaurianoJA, Ramalho JC, Lidon FC, Ce úmatos M (2006) Mechanisms of energy dissipationin peanut under water stress. Photosynthetica 44(3):404–410
- LazárD (2006) The polyphasic chlorophyll a fluorescence rise measured under highintensity of exciting light. Funct Plant Biol 33(1):9–30
- LazárD, Jablonsky J (2009) On the approaches applied in formulation of a kineticmodel of photosystem II: different approaches lead to different simulations ofthe chlorophyll a fluorescence transients. J Theor Biol 257(2):260–269
- LiG, Zhang ZS, Gao HY, Liu P, Dong ST, Zhang JW, Zhao B (2012) Effects ofnitrogen on photosynthetic characteristics of leaves from two differentstay-green corn (Zea mays L.) varieties at the grain-filling stage. CanJ Plant Sci 92:671–680
- LiuWJ, Chen YE, Tian WJ, Du JB, Zhang ZW, Xu F, Zhang F, Yuan S, Lin HH (2009)Dephosphorylation of photosystem II proteins and phosphorylation of CP29 inbarley photosynthetic membranes as a response to water stress. Biochim BiophysActa 1787(10):1238–1245
- LobellDB, Burke MB, Tebaldi C, Mastrandrea MD, Falcon WP, Naylor RL (2008)Prioritizing climate change adaptation needs for food security in 2030. Science319:607–610
- ŁobodaT, Kalaji MH (2007) Photosystem II of barley seedlings under cadmium and leadstress. Plant Soil Environ 53(12):511
- MalaspinaP, Giordani P, Faimali M, Garaventa F, Modenesi P (2014) Assessingphotosynthetic biomarkers in lichen transplants exposed under different lightregimes. Ecol Ind 43:126–131
- MallickN, Mohn F (2003) Use of chlorophyll fluorescence in metalstress research: acase study with the green microalga Scenedesmus. Ecotoxicol Environ Saf55(1):64–69
- MathurS, Mehta P, Jajoo A, Bharti S (2011a) Analysis of elevated temperature inducedinhibition of Photosystem II using Chl a fluorescence inductionkinetics. Plant Biology 13:1–6
- MathurS, Allakhverdiev SI, Jajoo A (2011b) Analysis of high temperature stress on thedynamics of antenna size and reducing side heterogeneity of Photosystem II inwheat leaves (Triticum aestivum). Biochim Biophys Acta 1807(1):22–29
- MathurS, Agrawal D, Jajoo A (2014) Photosynthesis: limitations in response to hightemperature stress. J Photochem Photobiol B Biol. doi: 10.1016/j.jphotobiol.2014.01.010
- MehtaP, Allakhverdiev SI, Jajoo A (2010a) Characterization of photosystem IIheterogeneity in response to high salt stress in wheat leaves (Triticumaestivum). Photosynth Res 105(3):249–255
- MehtaP, Jajoo A, Mathur S, Bharti S (2010b) Chlorophyll a fluorescence studyrevealing effects of high salt stress on Photosystem II in wheat leaves. PlantPhysiol Biochem 48(1):16–20
- MelisA, Homann PH (1976) Heterogeneity of the photochemical centers in system II ofchloroplasts. Photochem Photobiol 23:343–350
- MishraS, Dubey R (2005) Heavy metal toxicity induced alterations in photosyntheticmetabolism in plants. In: Pessarakli M (ed) Handbook of photosynthesis, vol 28.CRC Press, Boca Raton, pp 827–844
- MolassiotisA, Tanou G, Diamantidis G, Patakas A, Therios I (2006) Effects of 4-month Fedeficiency exposure on Fe reduction mechanism, photosynthetic gas exchange,chlorophyll fluorescence and antioxidant defense in two peach rootstocksdiffering in Fe deficiency tolerance. J Plant Physiol 163(2):176–185
- MurataN, Mohanty P, Hayashi H, Papageorgiou G (1992) Glycinebetaine stabilizes theassociation of extrinsic proteins with the photosynthetic oxygen-evolvingcomplex. FEBS Lett 296(2):187–189
- OukarroumA, Madidi SE, Schansker G, Strasser RJ (2007) Probing the responses of barleycultivars (Hordeum vulgare L.) by chlorophyll a fluorescence OLKJIPunder drought stress and rewatering. Environ Exp Bot 60(3):438–446
- OukarroumA, Schansker G, Strasser RJ (2009) Drought stress effects on photosystem Icontent and photosystem II thermotolerance analyzed using Chl afluorescence kinetics in barley varieties differing in their drought tolerance.Physiol Plant 137(2):188–199
- OukarroumA, El Madidi S, Strasser RJ (2012) Exogenous glycine betaine and proline play aprotective role in heat-stressed barley leaves (Hordeum vulgare L.): achlorophyll a fluorescence study. Plant Biosyst 146(4):1037–1043
- PapageorgiouGC, Govindjee (2011) Photosystem II fluorescence: slow changes—scaling from thepast. J Photochem Photobiol B 104(1–2):258–270
- RampinoP, Pataleo S, Gerardi C, Mita G, Perrotta C (2006) Drought stress response inwheat: physiological and molecular analysis of resistant and sensitivegenotypes. Plant Cell Environ 29(12):2143–2152
- RedillasMCFR, Jeong JS, Strasser RJ, Kim YS, Kim JK (2011) JIP analysis on rice (Oryzasativa cv Nipponbare) grown under limited nitrogen conditions. J Korean SocAppl Biol Chem 54:827–832
- RomanowskaE, Igamberdiev AU, Parys E, Gardeström P (2002) Stimulation of respiration byPb2+ in detached leaves and mitochondria of C3 and C4 plants.Physiol Plant 116(2):148–154
- RomanowskaE, Wróblewska B, Dro_ zak A, Siedlecka M (2006) High light intensity protects photosyntheticapparatus of pea plants against exposure to lead. Plant Physiol Biochem44(5):387–394
- SchanskerG, Srivastava A, Govindjee, Strasse RJ (2003) Characterization of the 820-nmtransmission signal paralleling the chlorophyll a fluorescence rise (OJIP)in pea leaves. Funct Plant Biol 30(7):785–796
- SchreiberU, Neubauer C, Klughammer C (1989) Devices and methods for room-temperaturefluorescence analysis. Philos Trans Royal Soc London B Biol Sci323(1216):241–251
- SchreiberU, Bilger W, Neubauer C (1994) Chlorophyll fluorescence as a nonintrusiveindicator for rapid assessment of in vivo photosynthesis. Ecophysiology ofphotosynthesis. Springer, Berlin, pp 49–70
- SchreiberU, Klughammer C, Kolbowski J (2012) Assessment of wavelength-dependent parametersof photosynthetic electron transport with a new type of multi-color PAMchlorophyll fluorometer. Photosynth Res 113(1–3):127–144
- SchweigerJ, Lang M, Lichtenthaler HK (1996) Differences in fluorescence excitationspectra of leaves between stressed and non-stressed plants. J Plant Physiol148(5):536–547
- SharkeyTD, Schrader SM (2006) High temperature stress. Physiology and molecularbiology of stress tolerance in plants. Springer, Berlin, pp 101–129
- SharmaP, Dubey RS (2005) Lead toxicity in plants. Brazilian Journal of PlantPhysiology 17:35–52
- SigfridssonKG, Berna ′t G, Mamedov F, Styring S (2004) Molecular interference of Cd2+with Photosystem II. Biochim Biophys Acta 1659(1):19–31
- Singh-TomarR, Mathur S, Allakhverdiev SI, Jajoo A (2012) Changes in PSII heterogeneity inresponse to osmotic and ionic stress in wheat leaves (Triticum aestivum). JBioenerg Biomembr 44:411–419
- SmethurstCF, Garnett T, Shabala S (2005) Nutritional and chlorophyll fluorescenceresponses of lucerne (Medicago sativa) to waterlogging and subsequent recovery.Plant Soil 270(1):31–45
- SrivastavaA, Strasser RJ (1995) How do land plants respond to stress temperature andstress light? Arch Sci Geneve 48:135–146
- SrivastavaA, Strasser RJ, Govindjee (1999) Greening of peas: parallel measurements of 77K emission spectra, OJIP chlorophyll a fluorescence transient, periodfour oscillation of the initial fluorescence level, delayed light emission, andP700. Photosynthetica 37:365–392
- StefanovD, Petkova V, Denev ID (2011) Screening for heat tolerance in common bean (Phaseolusvulgaris L.) lines and cultivars using JIP-test. Sci Hortic 128(1):1–6
- StirbetA (2013) Excitonic connectivity between photosystem II units: what is it, andhow to measure it? Photosynth Res 116:189–214
- StirbetA, Govindjee (2011) On the relation between the Kautsky effect (chlorophyll afluorescence induction) and Photosystem II: basics and applications of the OJIPfluorescence transient. J Photochem Photobiol B 104(1–2):236–257
- StirbetA, Govindjee (2012) Chlorophyll a fluorescence induction: a personalperspective of the thermal phase, the J–I–P rise. Photosynth Res 113:15–61
- StrasserRJ, Stirbet AD (1998) Heterogeneity of photosystem II probed by the numericallysimulated chlorophyll a fluorescence rise (O–J–I–P). Math Comput Simul48(1):3–9
- StrasserRJ, Srivastava A, Tsimilli-Michael M (2000) The fluorescence transient as atool to characterize and screen photosynthetic samples. In: Mohanty P, Yunus,Pathre (eds) Probing photosynthesis: mechanism, regulation and adaptation.Taylor and Francis, London, pp 443–480
- StrasserRJ, Tsimilli-Michael M, Srivastava A (2004) Analysis of the chlorophyll afluorescence transient. In: Papageorgiou G, Govindjee (eds) Advances inphotosynthesis and respiration. chlorophyll a fluorescence: a signatureof photosynthesis. Springer, Dordrecht, pp 321–362
- StrasserRJ, Tsimilli-Michael M, Srivastava A, Srivastava A (2005) Analysis of thechlorophyll a fluorescence transient. In: Papageorgiou GC, Govindjee(eds) Advances in photosynthesis and respiration. Chlorophyll afluorescence: a signature of photosynthesis. Kluwer Acad. Publ, Dordrecht, pp321–362
- StrasserRJ, Tsimilli-Michael M, Qiang S, Goltsev V (2010) Simultaneous in vivorecording of prompt and delayed fluorescence and 820-nm reflection changesduring drying and after rehydration of the resurrection plant Haberlearhodopensis. Biochimical BiophysicalActa 1797:1313–1326
- StraussA, Kru ¨ger G, Strasser RJ, Heerden PV (2006) Ranking of dark chillingtolerance in soybean genotypes probed by the chlorophyll a fluorescencetransient OJIP. Environ Exp Bot 56(2):147–157
- StrebP, Aubert S, Gout E, Feierabend J, Bligny R (2008) Cross tolerance toheavy-metal and cold-induced photoinhibiton in leaves of Pisum sativumacclimated to low temperature. Physiol Mole Biol Plants 14(3):185–193
- SuzukiK, Ohmori Y, Ratel E (2011) High root temperature blocks both linear and cyclicelectron transport in the dark during chilling of the leaves of rice seedlings.Plant Cell Physiology 52(9):1697–1707
- TomarRS, Jajoo A (2013) A quick investigation of the detrimental effects ofenvironmental pollutant polycyclic aromatic hydrocarbon fluoranthene on thephotosynthetic efficiency of wheat (Triticum aestivum). Ecotoxicology22(8):1313–1318
- TomarRS, Jajoo A (2014) Fluoranthene, a polycyclic aromatic hydrocarbon, inhibitslight as well as dark reactions of photosynthesis in wheat (Triticum aestivum).Ecotoxicol Environ Saf 109:110–115
- Tsimilli-MichaelM, Strasser RJ (2008) In vivo assessment of stress impact on plants’ vitality:applications in detecting and evaluating the beneficial role of Mycorrhizationon host plants. In: Varma A (ed) Mycorrhiza: state of the art, genetics and molecularbiology, eco-function, biotechnology, eco-physiology, structure andsystematics, vol 3rd. Springer, Berlin, pp 679–703
- Tsimilli-MichaelM, Strasser RJ (2013) The energy flux theory 35 years later: formulations andapplications. Photosynth Res 117(1–3):289–320. doi:10.1007/s11120-013-9895-1
- TubaZ, Saxena DK, Srivastava K, Singh S, Czebol S, Kalaji MH (2010) Chlorophyll afluorescence measurements for validating the tolerant bryophytes for heavymetal (Pb) biomapping. Curr Sci 98(11):1505–1508
- VanHeerden PD, Strasser RJ, Kru ¨ger GH (2004) Reduction of dark chilling stressin N2-fixing soybean by nitrate as indicated by chlorophyll a fluorescencekinetics. Physiol Plant 121:239–249
- VanHeerden PDR, Swanepoel JW, Kru ¨ger GHJ (2007) Modulation of photosynthesis bydrought in two desert scrub species exhibiting C3-mode CO2 assimilation.Environ Exp Bot 61(2):124–136
- WuC, Wang Z, Sun H, Guo S (2006) Effects of different concentrations of nitrogenand phosphorus on chlorophyll biosynthesis, chlorophyll a fluorescence,and photosynthesis in Larix olgensis seedlings. Front Forest China1(2):170–175
- WuX, Hong F, Liu C, Su M, Zheng L, Gao F, Yang F (2008) Effects of Pb2+on energy distribution and photochemical activity of spinach chloroplast.Spectrochimica Acta Part A Mol Biomol Spectrosc 69(3):738–742
- YamaneY, Kashino Y, Koike H, Satoh K (1997) Increases in the fluorescence Fo leveland reversible inhibition of Photosystem II reaction center by high-temperaturetreatments in higher plants. Photosynth Res 52:57–64
- YangX, Liang Z, Wen X, Lu C (2008) Genetic engineering of the biosynthesis ofglycinebetaine leads to increased tolerance of photosynthesis to salt stress intransgenic tobacco plants. Plant Mol Biol 66(1–2):73–86
- YangJ, Kong Q, Xiang C (2009) Effects of low night temperature on pigments, chl afluorescence and energy allocation in two bitter gourd (Momordica charantiaL.) genotypes. Acta Physiologiae Plantarum 31(2):285–293
- ZhangR, Sharkey TD (2009) Photosynthetic electron transport and proton flux undermoderate heat stress. Photosynth Res 100(1):29–43
- ZivcakM, Brestic M, Olsovska K, Slamka P (2008) Performance index as a sensitiveindicator of water stress in Triticum aestivum. Plant Soil Environ54:133–139
- ZivcakM, Brestic M, Balatova Z, Drevenakova P, Olsovska K, Kalaji MH, AllakhverdievSI (2013) Photosynthetic electron transport and specific photoprotectiveresponses in wheat leaves under drought stress. Photosynth Res 117:529–546
- ZivcakM, Kalaji MH, Shao HB, Olsovska K, Brestic M (2014). Photosynthetic proton andelectron transport in wheat leaves under prolonged moderate drought stress. JPhotochem Photobiol B Biol. doi: 10.1016/j.jphotobiol.2014.01.007
- ZivcakM, Olsovska K, Slamka P, Galambosova J, Rataj V, Shao HB, Brestic M (2014b)Application of chlorophyll fluorescence performance indices to assess the wheatphotosynthetic functions influenced by nitrogen deficiency. Plant Soil Environ60:210–215
- ZushiK, Kajiwara S, Matsuzoe N (2012) Chlorophyll a fluorescence OJIP transient as atool to characterize and evaluate response to heat and chilling stress intomato leaf and fruit. Sci Hortic 148:39–46
|
|